Exploring CRISPR: The Intersection of Science and Genetics
Written on
Chapter 1: The Fascination with Genetic Modification
As a child, I was captivated by the world of X-Men, where "mutants" with extraordinary abilities coexisted with ordinary humans. These mutants, possessing the “X-gene,” underwent transformations during puberty, granting them unique powers. Despite their remarkable gifts, they faced discrimination and were often marginalized by society. The X-Men, a coalition of these mutants, fought for harmony and acceptance between both groups.
If you were like me, you yearned for the day when science would turn such fictional powers into reality. While we may never witness the likes of Cyclops' powers, the advancement of genetic engineering, particularly through CRISPR, offers a glimpse into what is possible. CRISPR is often described as akin to a word processor for DNA, enabling the enhancement of living organisms' genomes, potentially including our own.
CRISPR is not the first method developed for genetic manipulation, yet its precision, affordability, and simplicity have made it a focal point in modern science. This article delves into the evolution and science behind CRISPR, starting from the basics of genetics and the human genome.
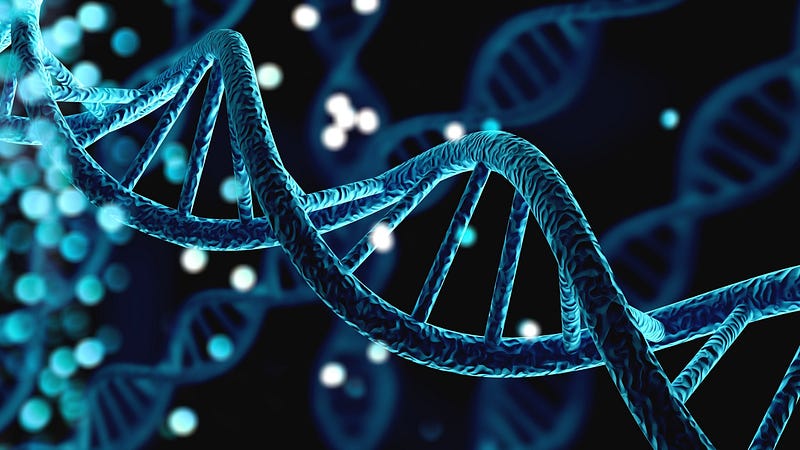
Chapter 2: Understanding Genetics
In your biology classes, you likely learned that characteristics such as eye color and height stem from genetic information encoded in your genome. This genome comprises deoxyribonucleic acid (DNA), which resides in nearly all your cells, remaining largely consistent except for occasional mutations. DNA provides the blueprint for growth, maintenance, and the transfer of genetic material to future generations, a principle that applies to all living organisms.
DNA consists of four bases: adenine (A), cytosine (C), thymine (T), and guanine (G). These bases form the rungs of a twisted ladder known as a double helix, with a sugar-phosphate backbone supporting the structure. In this configuration, A pairs with T and G pairs with C, forming base pairs. When cells divide, enzymes split the DNA strands, creating two identical copies for daughter cells, using base pairing as a guide.
But how does DNA influence your identity? Imagine DNA as a secret language where the sequence of letters provides instructions for synthesizing proteins, which are vital for numerous bodily functions, from digestion to sensory perception.
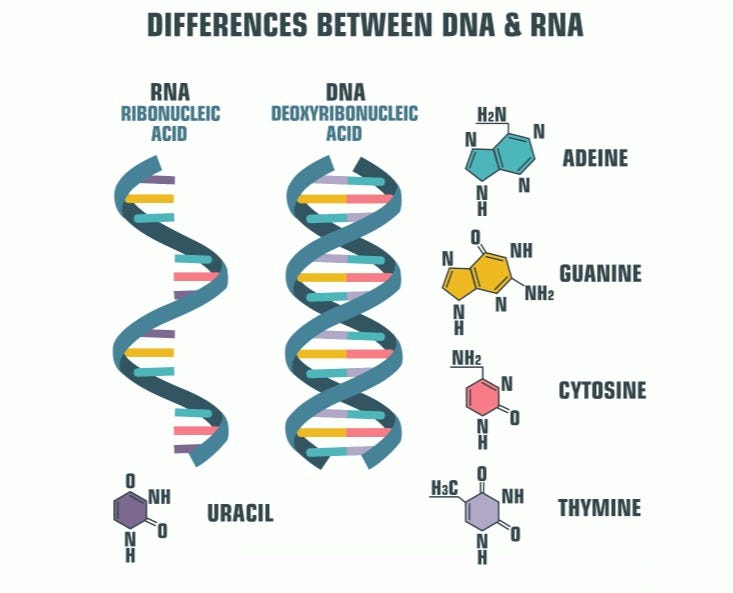
To synthesize proteins based on DNA's directives, an intermediary molecule known as ribonucleic acid (RNA) is necessary. RNA is synthesized from DNA through transcription, utilizing the bases A, C, U, and G, replacing thymine with uracil. Acting as a messenger, RNA transports information from the nucleus to various cellular locations for protein production.
During translation, specific DNA segments generate long RNA strands, which serve as templates for constructing proteins. The ribosome interprets the RNA sequence, with three-letter combinations, or codons, corresponding to one of 20 amino acids. While there are 64 potential codons, only 20 amino acids exist since multiple codons can code for a single amino acid. This foundational process, termed the central dogma of molecular biology, describes how DNA translates into the proteins that constitute all life forms.
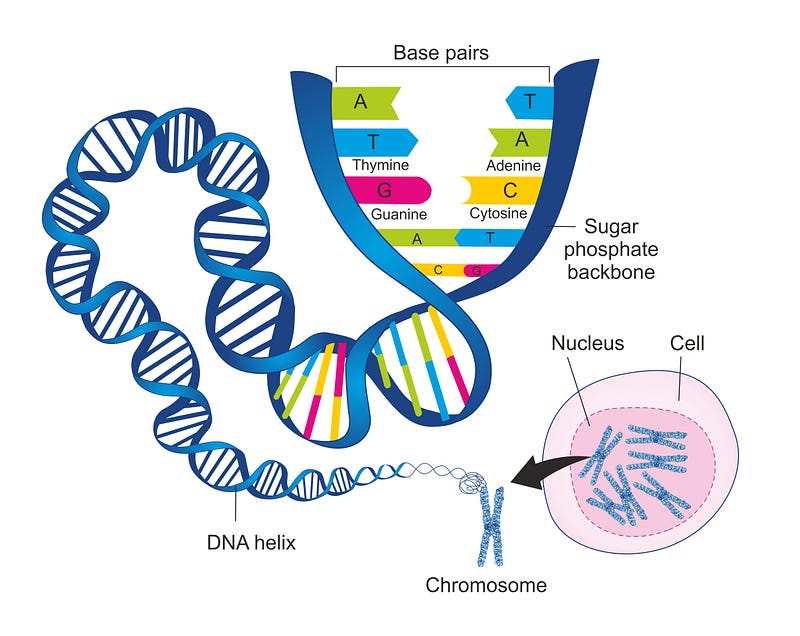
Chapter 3: The Human Genome
The human genome encompasses approximately 3.2 billion base pairs, coding for around 20,000 proteins. Organized into 23 distinct chromosomes, each spanning 50 to 250 million base pairs, humans inherit 23 chromosomes from each parent, totaling 46 per cell. Nearly every cell in the body contains a complete set of chromosomes.
Mutations, or changes in the DNA sequence, are part of our genetic makeup. One simple mutation, known as substitution, occurs when one base is replaced by another. While seemingly minor, such mutations can lead to severe health issues. For instance, sickle cell anemia arises from a substitution in the beta-globin gene, altering a single amino acid in hemoglobin and leading to significant health complications.
The influence of our DNA sequence on our biology and health is profound, prompting global initiatives like the Human Genome Project. Initiated in the 1970s, this project utilized emerging DNA sequencing technologies to map the human genome. By 2001, the first draft was published, marking a pivotal achievement in understanding our genetic instructions.
The Human Genome Project concluded in 2003, with 92% of the genome sequenced. Remaining regions posed challenges due to highly repetitive DNA, but advancements in sequencing technology have since enabled the completion of the human genome in 2022.
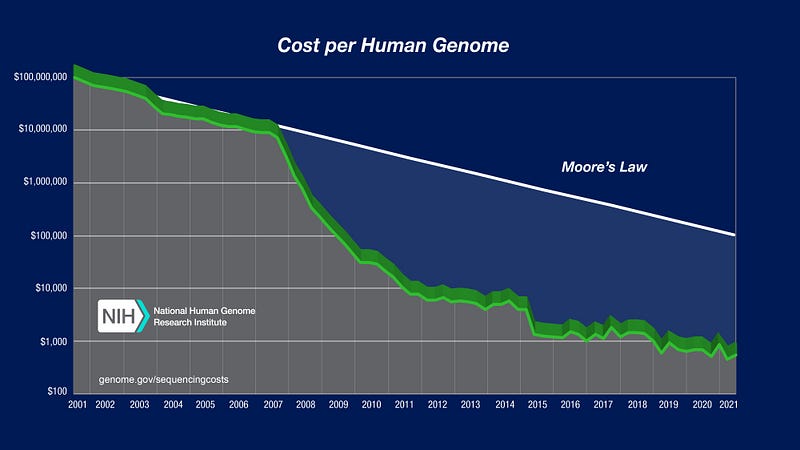
The initial sequencing of the human genome cost over $3 billion, but technological advances have drastically reduced this price. Currently, individuals can have their genomes sequenced for under $1,000, with expectations that this will soon drop to around $100.
With insights from the Human Genome Project and advancing sequencing techniques, scientists have identified over 4,000 mutations linked to genetic disorders, aiding in personalized treatment strategies and revealing relationships between gene variants and various traits. However, genome sequencing primarily serves as a diagnostic tool. What if there existed an efficient, cost-effective method to modify DNA?
Chapter 4: Enter CRISPR
This is where CRISPR emerges. An abbreviation for “Clustered Regularly Interspaced Short Palindromic Repeats,” CRISPR is the latest innovation in gene editing, revolutionizing the scientific landscape. Initially, researchers studied CRISPR to understand bacterial defenses against viruses. They discovered that bacteria possess short, repetitive DNA sequences interrupted by unique segments, forming the basis of the CRISPR system.
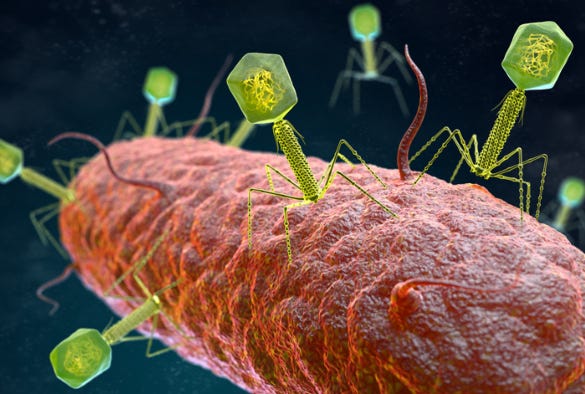
Bacteriophages, viruses that infect bacterial cells, are ubiquitous, with estimates of around 10³¹ bacteriophages on Earth. These viruses invade bacterial cells, injecting their genetic material and commandeering the host's resources to replicate until the cell ruptures, releasing new phages to infect neighboring cells.
To combat this, bacteria have evolved the CRISPR system as a form of adaptive immunity. During an infection, they incorporate fragments of viral DNA into their own genome, which they later use to identify and eliminate the same viruses in subsequent encounters.
When re-infected, bacteria convert the CRISPR array into RNA strands, which guide Cas proteins to the corresponding viral DNA sequences, allowing for precise cuts in the target DNA.
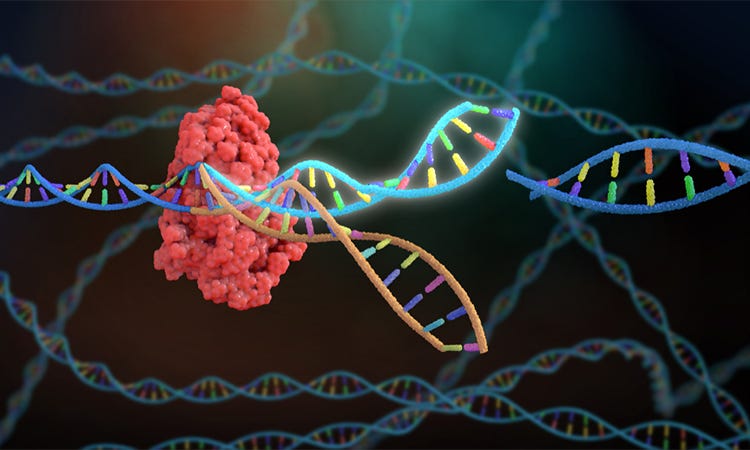
The Cas9 protein, a crucial component of this system, opens the DNA double helix and targets specific sequences for cutting. When researchers initially identified Cas9 in bacterial defenses, they recognized its potential for editing other DNA sequences, including those of humans.
This discovery has led to a programmable system capable of targeting any 20-letter DNA sequence. By integrating tracrRNA and guide RNA, scientists have enhanced the efficacy of the CRISPR-Cas9 system.
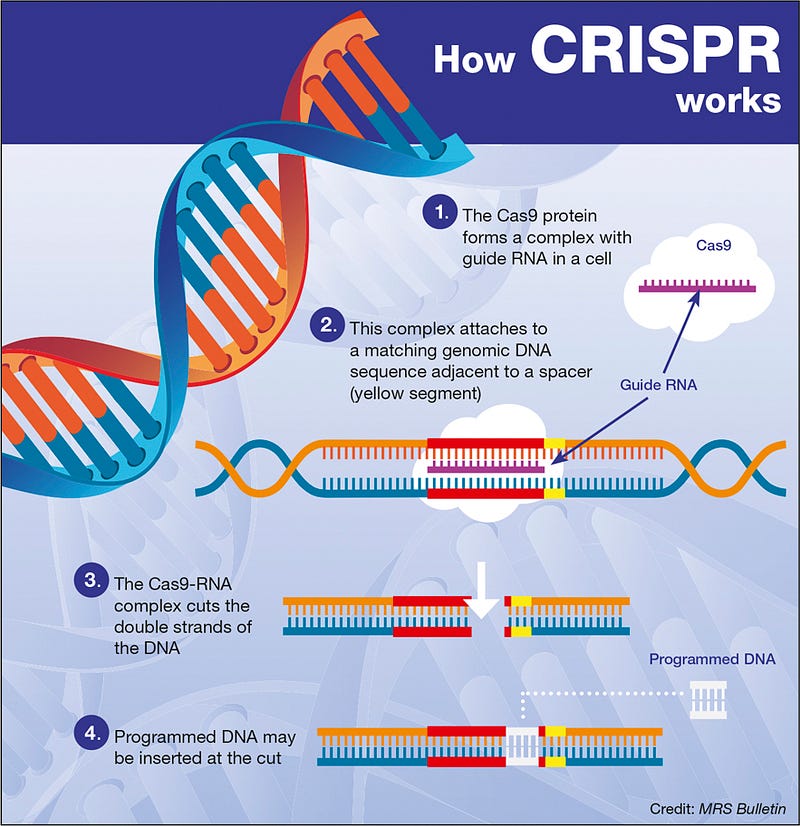
In practical terms, the CRISPR-Cas9 system allows for the customization of RNA to match a specific gene sequence. Once Cas9 and guide RNA are introduced into a target cell, the system makes precise cuts in the DNA, which the cell then repairs, potentially incorporating new genetic material.
This capability enables not only minor alterations in the DNA but also the possibility of larger changes, such as gene swaps or deletions. Consequently, when new DNA instructions are integrated, they influence gene expression and protein synthesis.
The implications of CRISPR are vast, ranging from curing genetic diseases to engineering crops that can withstand drought. Its potential raises ethical questions about human genetic modification and the creation of “designer babies.” Ultimately, CRISPR empowers humanity to reshape not just our environment but also our very selves. What are your thoughts on this transformative technology? Feel free to share in the comments!
Enjoyed this article? Check out these related topics:
- A “De-Extinction” Company Plans to Revive the Already Extinct Tasmanian Tiger — Can genetic engineering and reproductive technology de-extinct the Tasmanian Tiger?
- Nanotechnology vs. Cancer: How Liposomes Have Revolutionized Chemotherapy — Cancer drugs that are more effective and have fewer side effects.
- Artificial Intelligence Reveals the Entire Protein Structure Universe — Soon, you’ll be able to obtain the 3D structure of any known protein simply by querying a database.
References:
- Doudna, Jennifer A., and Samuel H. Sternberg. A Crack in Creation: Gene Editing and the Unthinkable Power To Control Evolution. Mariner, 2018.